Sleep is among the most enduring mysteries in biology. We spend a third of our lives asleep, sleep disorders are common, and sleep is evolutionarily conserved, yet mechanisms that underlie this behavioral state remain poorly understood. We are taking a new approach to this problem by exploiting useful features of zebrafish to answer a basic question: What genetic and neuronal mechanisms underlie sleep? We are addressing this question using a combination of genetics, behavioral assays, optogenetic and chemogenetic perturbations, whole-brain imaging, and electrophysiology, which together are providing new insights into mechanisms that underlie sleep and wakefulness.
A model for sleep/wake regulation
Sleep is regulated by a homeostatic process (process S) that reflects internal cues of sleep need and a circadian process (process C) that is entrained by environmental cues and restricts sleep to the appropriate time of the day/night cycle. Sleep is also directly and rapidly affected by light and dark, a phenomenon known as masking. We are using zebrafish to uncover mechanisms that underlie each of these processes. To do so, we have developed tools and assays to study zebrafish sleep, including high-throughput assays for sleep, sleep deprivation, and arousal threshold, which have allowed us to perform screens to identify genes and drugs that affect sleep. We have also developed non-invasive and large-scale optogenetic and chemogenetic assays to ask how perturbation of specific neurons affects neuronal activity and behavior. Finally, we are using two-photon light sheet microscopy to perform whole-brain imaging in order to comprehensively and efficiently identify neuronal mechanisms that underlie sleep and wakefulness.
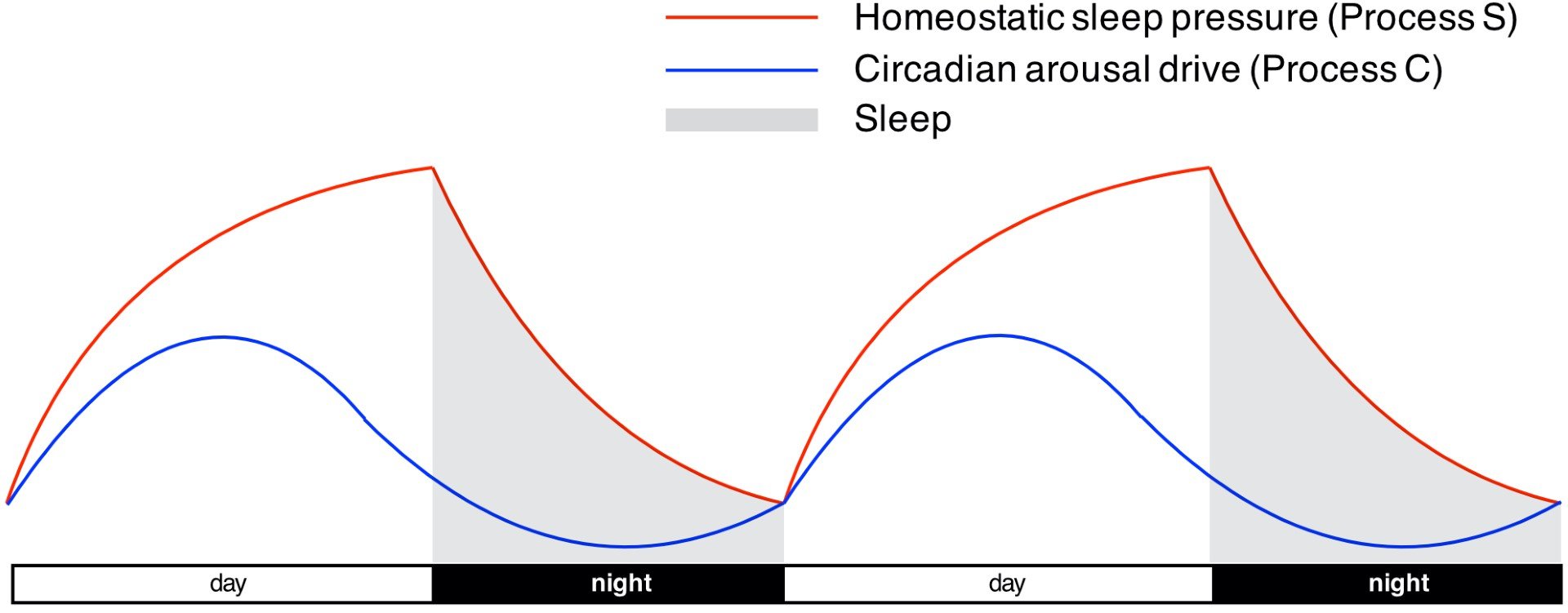
Homeostatic regulation of sleep
We have identified a genetic and neuronal circuit that plays a key role in homeostatic regulation of sleep. This is based on our discovery that a small population of neurons in the hypothalamus that expresses the neuropeptide NPVF functions to promote sleep. Stimulation of these neurons or overexpression of NPVF results in increased sleep, while ablation of these neurons or loss of NPVF signaling results in decreased sleep. In subsequent studies, we found that epidermal growth factor receptor (EGFR) signaling promotes sleep by activating NPVF neurons, and that NPVF neurons, in turn, promote sleep by activating a population of neurons in the serotonergic raphe. Loss of EGFR signaling or raphe neurons results in reduced rebound sleep following sleep deprivation, indicating that the EGFR-NPVF-raphe pathway promotes homeostatic regulation of sleep. We are now working to identify genes and neurons that act upstream and downstream of this pathway to promote sleep, and also to understand how this pathway regulates sleep homeostasis. One potential clue is based on our observation that raphe neurons fire at higher rates during wake compared to sleep in both zebrafish and mice, suggesting that the raphe might act to build homeostatic sleep pressure by measuring the duration and intensity of waking. These findings are likely to be directly relevant to humans, since genetic variation in the EGFR and serotonin pathways are associated with human sleep disorders.
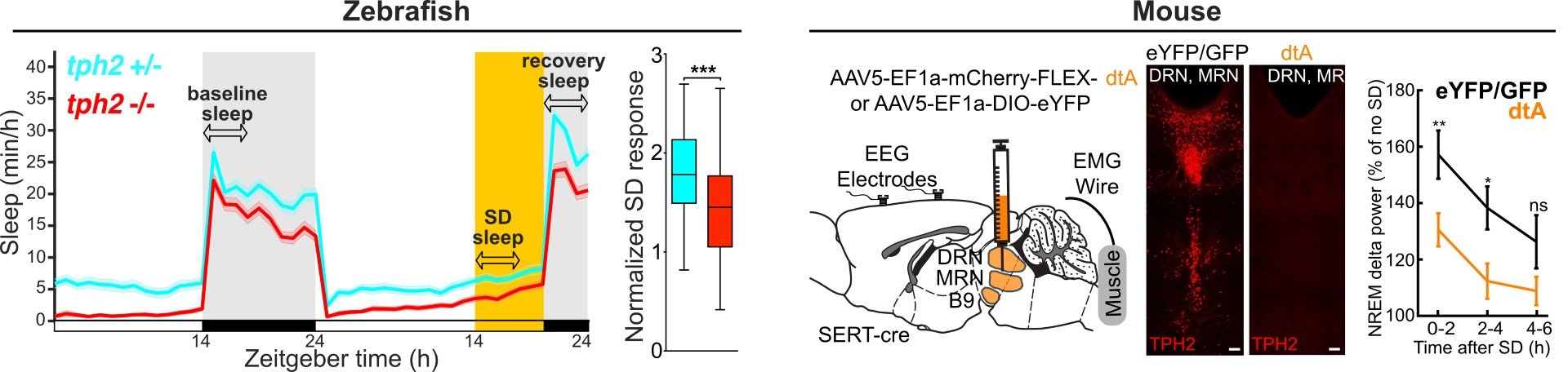
Circadian regulation of sleep
Mechanisms that regulate the circadian clock are well-established, but prior to our work the factors that transmit circadian information to regulate sleep, and thus mediate process C, were unknown. Melatonin is a good candidate factor because its synthesis is regulated by the circadian clock, and it can induce sleep in diurnal species, including zebrafish and humans. However, the role of melatonin in sleep is controversial for three reasons. First, most lab mouse strains produce little or no melatonin, yet show circadian control of sleep. Second, melatonin synthesis peaks at night in both diurnal and nocturnal animals, and melatonin does not induce sleep in nocturnal animals, suggesting that melatonin only regulates sleep in diurnal animals. Third, studies in diurnal species have failed to show a consistent role for melatonin in sleep. However, these studies used crude pinealectomy methods, and distinct phenotypes may result from differences in the pinealectomy procedure in different species. The pineal gland also likely has functions other than melatonin production, so pinealectomy may have effects that obscure melatonin-dependent phenotypes. To avoid these confounds we used genetics and the diurnal zebrafish model to explore the function of melatonin in sleep. We found that zebrafish aanat2 mutants, which fail to synthesize melatonin, have normal circadian rhythms, but completely lack circadian regulation of sleep, indicating that melatonin acts downstream of the circadian clock to promote sleep, and thus mediates process C. We are currently studying the mechanisms through which melatonin regulates sleep.
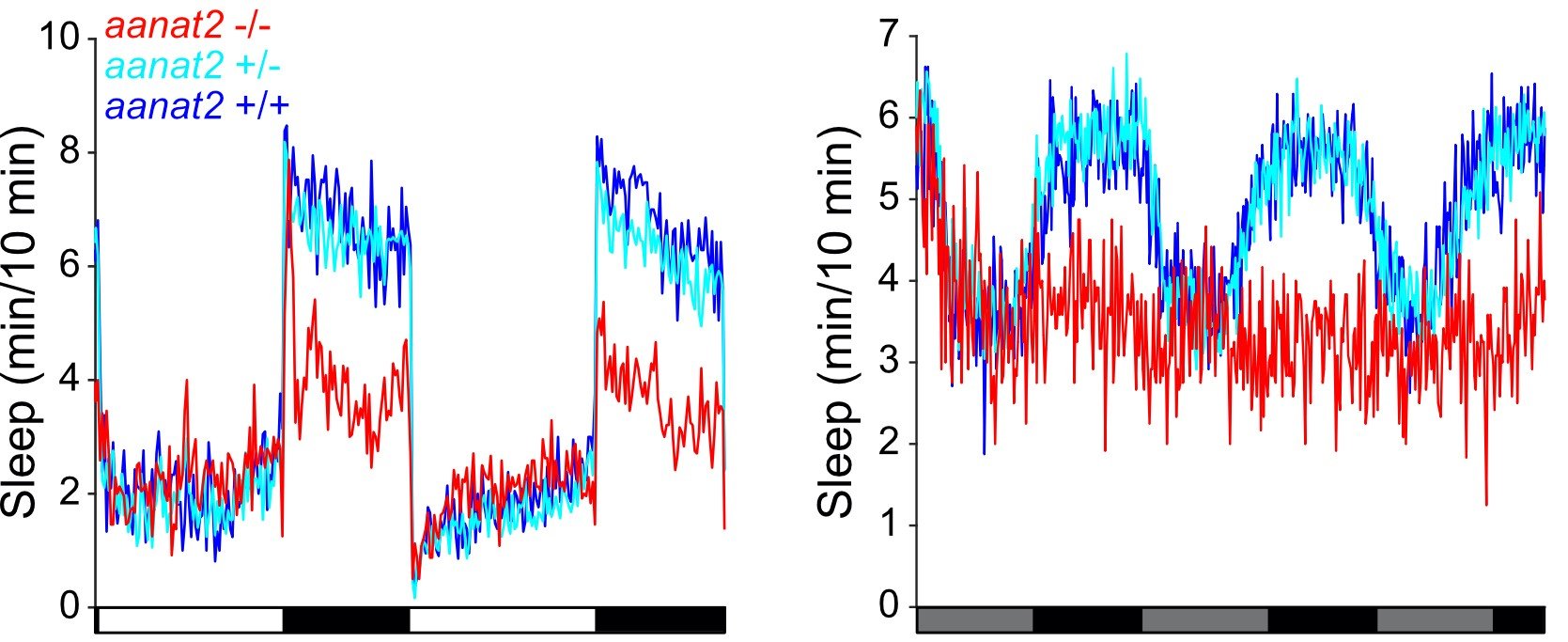
Light-dependent regulation of sleep
Animals that lack circadian rhythms have normal sleep patterns when maintained in normal light/dark conditions because (e.g. for diurnal animals) light promotes wake during the day and dark promotes sleep at night. Thus, light and dark can "mask" circadian phenotypes by directly and rapidly inducing wake and sleep, respectively, in diurnal animals (and have the opposite effects in nocturnal animals). Masking is widespread in the animal kingdom, but mechanisms that underlie masking downstream of light detection in the retina are largely unknown. These mechanisms are likely to be at least partially distinct in diurnal and nocturnal animals, highlighting the need to use diurnal animals to gain insights relevant to humans. Masking is clinically relevant because much of the sleep disruption observed in modern society results from exposure to artificial light at night, which suppresses sleep both directly via masking and indirectly via the circadian system. We discovered that the hypothalamic neuropeptide prokineticin 2 suppresses masking, in part by regulating expression of galanin in the zebrafish homolog of the mammalian ventrolateral preoptic nucleus, a gene and neuronal population known to regulate zebrafish and mammalian sleep. We are now exploring the mechanisms through which light/dark cues and prokineticin 2 regulate masking.
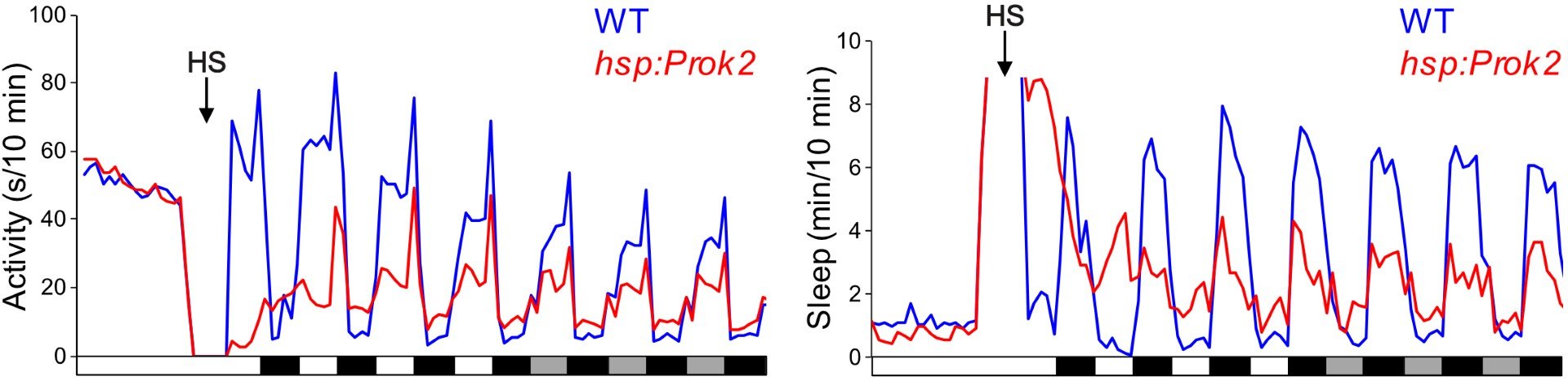
Genes associated with human sleep disorders
Studies using animal models have identified several genetic and neuronal circuits that regulate sleep, including genes that we identified by performing genetic screens in zebrafish. However, with the exceptions of narcolepsy and some circadian disorders, the genes and mechanisms that underlie most human sleep disorders are unknown. Recent human genome-wide association studies have identified many genomic loci that contain genetic variants that show significant association with human sleep traits and sleep disorders. However, in most cases the relevant gene within each locus is unknown, and must be experimentally determined. Doing so using rodents is challenging due to the high cost of generating and testing many mutant lines. In contrast, many zebrafish lines can be rapidly and inexpensively generated and behaviorally tested. We are using CRISPR/Cas9 to mutate the zebrafish orthologs of a large number of candidate human sleep disorder genes. This work has identified several novel sleep regulators, and we are performing mechanistic studies to determine how each gene regulates sleep.

Genes and mechanisms that underlie autism
Autism spectrum disorder (ASD) is caused by both environmental and genetic factors, with the genetic contribution estimated at 60-80%. Several labs have recently identified a large number of candidate ASD risk genes. The functions of most of these genes are unknown, so a crucial and necessary next step in order to determine if each gene is likely to be associated with ASD is to mutate each gene in a model organism and test for neurodevelopmental and behavioral phenotypes. The zebrafish is an outstanding animal model for this purpose because mechanisms that underlie neuronal development in zebrafish are broadly conserved with those of mammals, and because ASD is associated with several behaviors that can be modeled in zebrafish, including disrupted sleep, susceptibility to seizures, abnormal responses to stimuli, and disrupted social interactions. We recently found that mutation of the candidate ASD risk gene nr3c2 in zebrafish results in disrupted sleep at night and disrupted social interactions, suggesting that this gene is likely to be associated with ASD. We are currently determining the mechanistic basis for these phenotypes in nr3c2 mutants, and also for many other candidate ASD risk genes whose mutation in zebrafish results in behavioral phenotypes. Rather than assess ASD-risk genes one at a time, we are accelerating progress towards mechanistic understanding via high-throughput assays and analyses.