PASADENA, Calif.--Tiny, lightweight fruit flies need to know when it's windy out so they can steady themselves and avoid being knocked off their feet or blown off course. But how do they figure out that it's time to hunker down? According to a team led by California Institute of Technology (Caltech) scientists reporting in this week's issue of the journal Nature, the flies have evolved a specialized population of neurons in their antennae that let them know not only when the wind is blowing, but also the direction from which it is coming.
The behavior of fruit flies in the face of a stiff breeze is remarkable in and of itself, notes David J. Anderson, the Roger W. Sperry Professor of Biology at Caltech, and a Howard Hughes Medical Institute (HHMI) Investigator. "We discovered that you can stop a fly dead in its tracks by blowing a gentle stream of air over it," he explains, adding that the flies' immobility is so complete, you could pick one up with a pair of chopsticks as long as a steady stream of wind was passing over the insect. Once the wind stops blowing, however, the flies immediately start walking around again. [EDITORS: Video of this behavior in the flies is available on request.]
But the response is also of interest from a scientific point of view, because it represents a fairly simple, innate defensive response that scientists can begin to tease apart in order to understand just how such behaviors are programmed in our genes. "It's more than just stupid pet tricks with fruit flies," Anderson says.
"We quickly realized that it would be interesting to ask just how the wind acts on the flies to make them stop walking. How do they sense the wind? How do they transfer that message to their brain so they know to stop moving while the wind is blowing?"
As it turns out, fruit flies are unusual in how they sense wind. Other insects have sensory hairs that stand up from the cuticle--or outer body wall--and, when blown about by a passing wind, trigger a neural response. The fruit flies, on the other hand, use their antennae to detect a breeze and its general direction, based on how the antenna moves in the breeze.
"This posed a bit of a puzzle for us," Anderson explains. "It's been long assumed that the main function of the neurons in the antennae was hearing."
And that is at least one of the antennae's functions. The flies' antennae detect nearby sounds--like the male's courtship song--that cause vibrations in the air, a bit like ripples in a pond after a rock has been thrown. Those vibrations twist the antennae slightly, exciting the neurons within.
Wind, on the other hand, is not a regularly oscillating wave; instead, it's a steady stream of air particles moving past the fly from various directions. The antennae move in the wind, but they don't twist rapidly back and forth as they do in response to sound.
Says Anderson: "What we wanted to understand was, how can flies tell the difference between sound and wind using the same sensory organ?"
There were two possible answers to this question. The first was that a fly's antennae are equipped with a single, versatile type of neuron that changes its firing pattern depending on whether it's detecting sound or wind, and that the differences in that firing pattern are picked up and somehow decoded by the fly's brain.
The other possibility, says Anderson, was that a fly's antennae contain two distinctly different populations of neurons--one that responds to oscillating air to detect sound, and another that responds to flowing air particles to detect wind.
The right answer? Number two. By selectively knocking out subsets of neurons, Anderson's graduate student Suzuko Yorozu was able to show that Johnston's organ--an area in a fruit fly antenna where sound detection is known to occur--does indeed contain at least two entirely separate groups of neurons. She also showed that each neuron type detects only one type of stimulus (sound for one; wind for the other), and that each sends its message to a distinct and separate area of the brain.
"The sound-sensitive neurons are preferentially activated by small movements of the antenna that are oscillatory in nature, firing only when the antenna twists, and turn off quickly," says Anderson. "The neurons that respond to wind, on the other hand, turn on when the antenna is pushed by air flow, and they stay on until the wind stops blowing." In other words, says Yorozu, "the intrinsic properties of these neurons are very different."
The end result of these separate pathways is that the flies exhibit absolutely distinct types of behaviors, with the sound-detecting neurons leading to behaviors like copulation (in the case of the courtship song), while the wind-detecting neurons prompt flies to come to a dead stop for safety's sake when air is blowing past with any real speed.
In addition to Anderson and Yorozu, other authors on the Nature paper, "Distinct sensory representations of wind and near-field sound in the Drosophila brain," include Caltech and HHMI postdoctoral scholar Allan Wong, Caltech visiting associate Brian Fischer, Caltech postdoctoral scholar Heiko Dankert, Maurice Kernan from SUNY Stony Brook, Azusa Kamikouchi from the University of Tokyo and the University of Cologne, and Kei Ito from the University of Cologne.
This work was supported by a grant from the National Science Foundation.
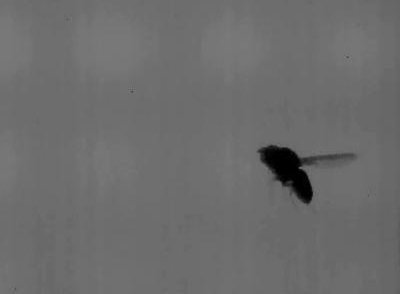
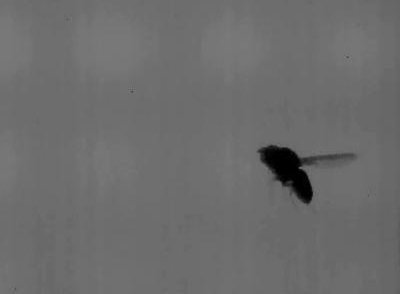