Gene therapy is a powerful developing technology that has the potential to address myriad diseases. For example, Huntington's disease, a neurodegenerative disorder, is caused by a mutation in a single gene, and if researchers could go into specific cells and correct that defect, theoretically those cells could regain normal function.
A major challenge, however, has been creating the right "delivery vehicles" that can carry genes and molecules into the cells that need treatment, while avoiding the cells that do not.
Now, a team led by Caltech researchers has developed a gene-delivery system that can specifically target brain cells while avoiding the liver. This is important because a gene therapy intended to treat a disorder in the brain, for example, could also have the side effect of creating a toxic immune response in the liver, hence the desire to find delivery vehicles that only go to their intended target. The findings were shown in both mouse and marmoset models, an important step towards translating the technology into humans.
A paper describing the new findings appears in the journal Nature Neuroscience on December 9. The research was led by Viviana Gradinaru (BS '05), professor of neuroscience and biological engineering, and director of the Center for Molecular and Cellular Neuroscience.
The key to this technology is the use of adeno-associated viruses, or AAVs, which have long been considered promising candidates for use as delivery vehicles. Over millions of years of evolution, viruses have evolved efficient ways to gain access into human cells, and for decades researchers have been developing methods to harness viruses' Trojan-Horse-like abilities for human benefit.
AAVs are made up of two major components: an outer shell, called a capsid, that is built from proteins; and the genetic material encased inside the capsid. To use recombinant AAVs for gene therapy, researchers remove the virus's genetic material from the capsid and replace it with the desired cargo, such as a particular gene or coding information for small therapeutic molecules.
"Recombinant AAVs are stripped of the ability to replicate, which leaves a powerful tool that is biologically designed to gain entrance into cells," says graduate student David Goertsen, a co-first author on the paper. "We can harness that natural biology to derive specialized tools for neuroscience research and gene therapy."
The shape and composition of the capsid is a critical part of how the AAV enters into a cell. Researchers in the Gradinaru lab have been working for almost a decade on engineering AAV capsids that cross the blood-brain barrier (BBB) and to develop methods to select for and against certain traits, resulting in viral vectors more specific to certain cell types within the brain.
In the new study, the team developed BBB-crossing capsids, with one in particular —AAV.CAP-B10—that is efficient at getting into brain cells, specifically neurons, while avoiding many systemic targets, including liver cells. Importantly, both neuronal specificity and decreased liver targeting was shown to occur not just in mice, a common research animal, but also in laboratory marmosets.
"With these new capsids, the research community can now test multiple gene therapy strategies in rodents and marmosets and build up evidence necessary to take such strategies to the clinic," says Gradinaru. "The neuronal tropism and decreased liver targeting we were able to engineer AAV capsids for are important features that could lead to safer and more effective treatment options for brain disorders."
The development of an AAV capsid variant that works well in non-human primates is a major step towards the translation of the technology for use in humans, as previous variants of AAV capsids have been unsuccessful in non-human primates. The Gradinaru lab's systematic in vivo approach, which uses a process called directed evolution to modify AAV capsids at multiple sites has been successful in producing variants that can cross the BBBs of different strains of mice and, as shown in this study, in marmosets.
"Results from this research show that introducing diversity at multiple locations on the AAV capsid surface can increase transgene expression efficiency and neuronal specificity," says Gradinaru. "The power of AAV engineering to confer novel tropisms and tissue specificity, as we show for the brain versus the liver, has broadened potential research and pre-clinical applications that could enable new therapeutic approaches for diseases of the brain."
The paper is titled "AAV capsid variants with brain-wide transgene expression and decreased liver targeting after intravenous delivery in mouse and marmoset." Goertsen; Nicholas Flytzanis (PhD '18), the former scientific director of the CLARITY, Optogenetics and Vector Engineering Research (CLOVER) Center of Caltech's Beckman Institute; and former Caltech postdoctoral scholar Nick Goeden are co-first authors. Additional coauthors are graduate student Miguel Chuapoco, and collaborators Alexander Cummins, Yijing Chen, Yingying Fan, Qiangge Zhang, Jitendra Sharma, Yangyang Duan, Liping Wang, Guoping Feng, Yu Chen, Nancy Ip, and James Pickel.
Funding was provided by the Defense Advanced Research Projects Agency, the National Institutes of Health, and the National Sciences and Engineering Research Council of Canada.
Flytzanis, Goeden, and Gradinaru are co-founders of Capsida Biotherapeutics, a Caltech-led startup company formed to develop AAV research into therapeutics.
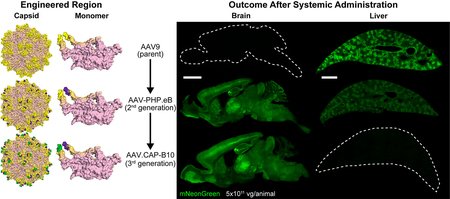
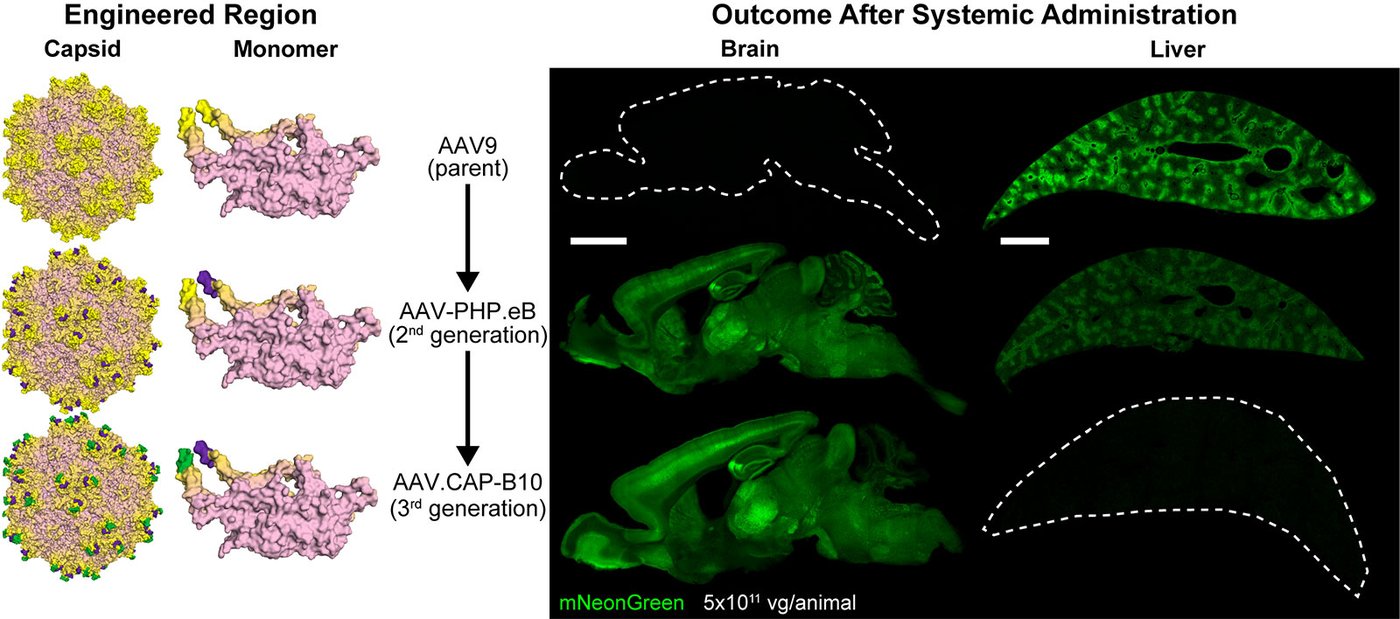
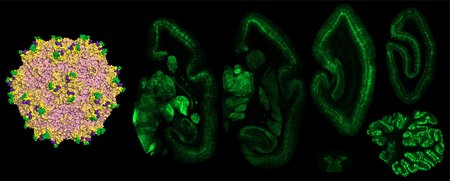
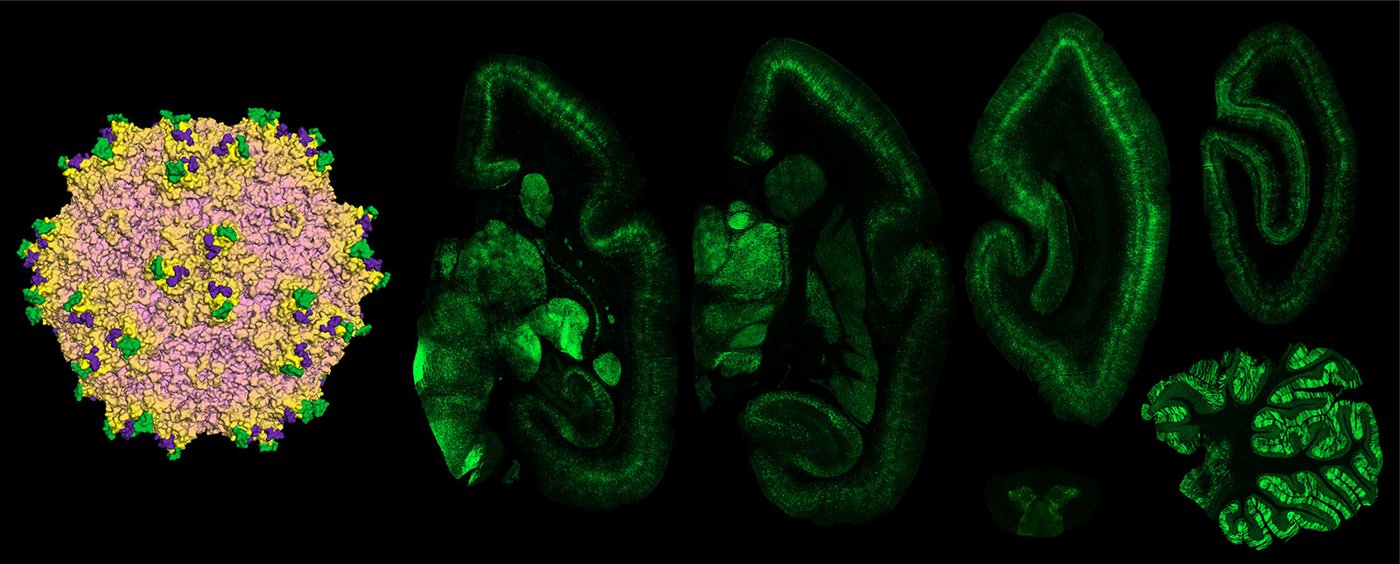